Aqueous Lubrication with Polymer Brushes
Introduction
Oil-based lubrication is widely used for many engineering surfaces at different contact scales to reduce friction, wear and enhance the lifetime of materials. Oil-based lubricants lead to lubricant retention in the contact, even at high contact pressures, and the avoidance of direct contact between the shearing surfaces, due the high viscosity and pressure-viscosity coefficient of the oils. However, oil-based lubricants generate pollutants and harmful contaminants and thus there is constant search for alternative green lubricants that are renewable and environmentally friendly. Lubrication in an aqueous medium has attracted significant attention over the last decade, owing to its environmental compatibility and ready availability of the lubricant, and has potential applications in food, biomedical and pharmaceutical industries. Despite the enormous advantages of water-based lubrication, it is rarely applied as a lubricant due to corrosive nature of water towards ferrous materials and also due its low pressure-coefficient of viscosity. Water at high contact pressures is immediately squeezed out of the contact area, promoting direct asperity-asperity contact and further resulting in significant friction in the boundary lubrication regime.
Nevertheless, lubrication in nature is exclusively achieved with water, which successfully resolves the above-mentioned limitations by employing brush-assisted aqueous lubrication, e.g. in synovial joint lubrication [1,2]. These heavily hydrated and compliant structures enable extraordinary tribological properties for an enduring time. The water molecules themselves can contribute to the resulting friction by becoming incorporated into the brush-structured proteoglycan to provide a ‘fluid-like’ cushioning layer [3].
Inspired by this approach, it is highly desirable to design and fabricate synthetic waterborne films with brushes structure mimicking the natural lubrication mechanism, in an attempt to understand the origins of the very efficient lubrication in living joints, and potentially enabling the application of aqueous lubrication in relevant industries.
References
Boundary Lubrication Properties of Poly(L-lysine)-g-poly(ethylene glycol) (PLL-g-PEG) as an Additive in Aqueous Media
Background
Low µ in well-designed systems
Natural joints (all aq. based) show frictional coefficients as low as the best man-made systems (µ=0.001)
Boundary lubrication in natural systems: what mechanism?
(Glyco)Proteins seems to be involved, although lipids have also been shown to display boundary lubrication ability.
High-pressure problem
Water, unlike oils, has a low pressure-dependence of viscosity, rendering EHL difficult or impossible. Need for designed surfaces to compensate this effect. Nature solves this problem with the sophisticated cartilage design.
Environmental/safety issues
Clear environmental/safety advantages of water over oil. Possibility for applications in the food, textile, or mining industries.
Poly(L-lysine)-g-poly(ethylene glycol) (PLL-g-PEG)
PLL backbone
MW: 20,000 to 350,000 D
-positively charged at pH<10 (R= –NH3+)
-approximate length of backbone: 90 to 1000 nm
PEG side chain
MW: 2000 to 5000 D
-adsorbs water and has properties similar to water
-protein resistant
-approximate length of side chain: 20 nm
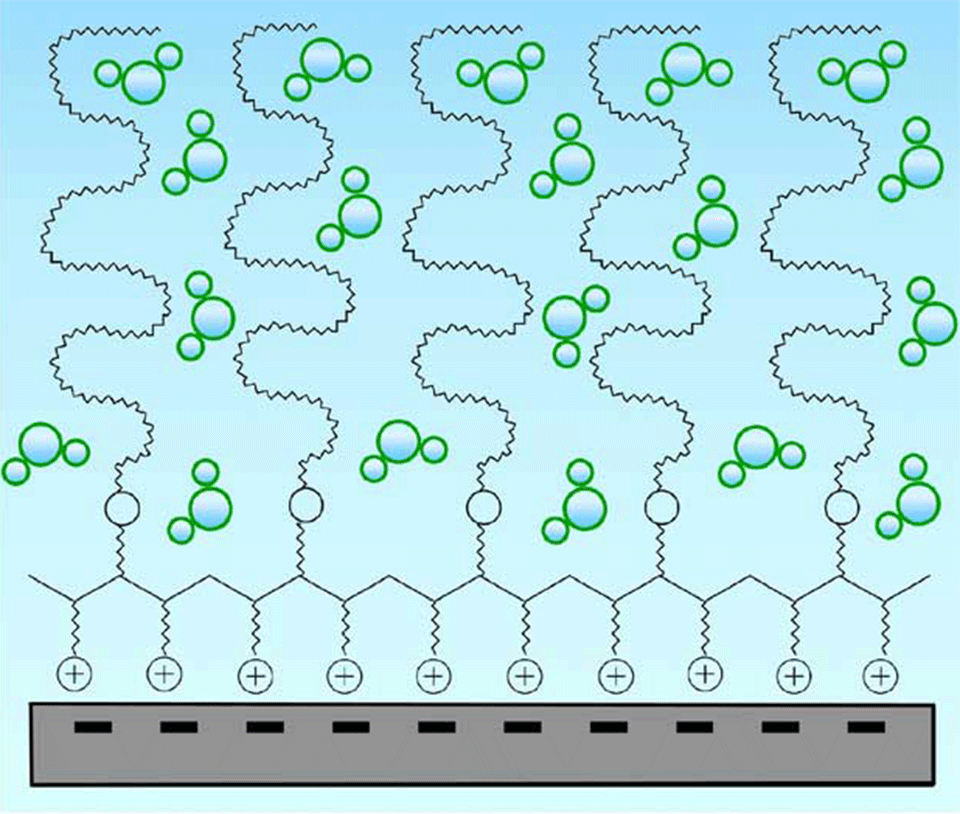
Some characteristic results
We have employed the tribo-pair composed of steel and glass as a model system to investigate the lubrication properties of the PLL-g-PEG at macroscopic scale contact. Combined study of Ultra-thin film interferometry (see Figure 3) and Mini-traction machine (MTM, see Figure 4) allows the film thickness and friction measurements at pure rolling contact.
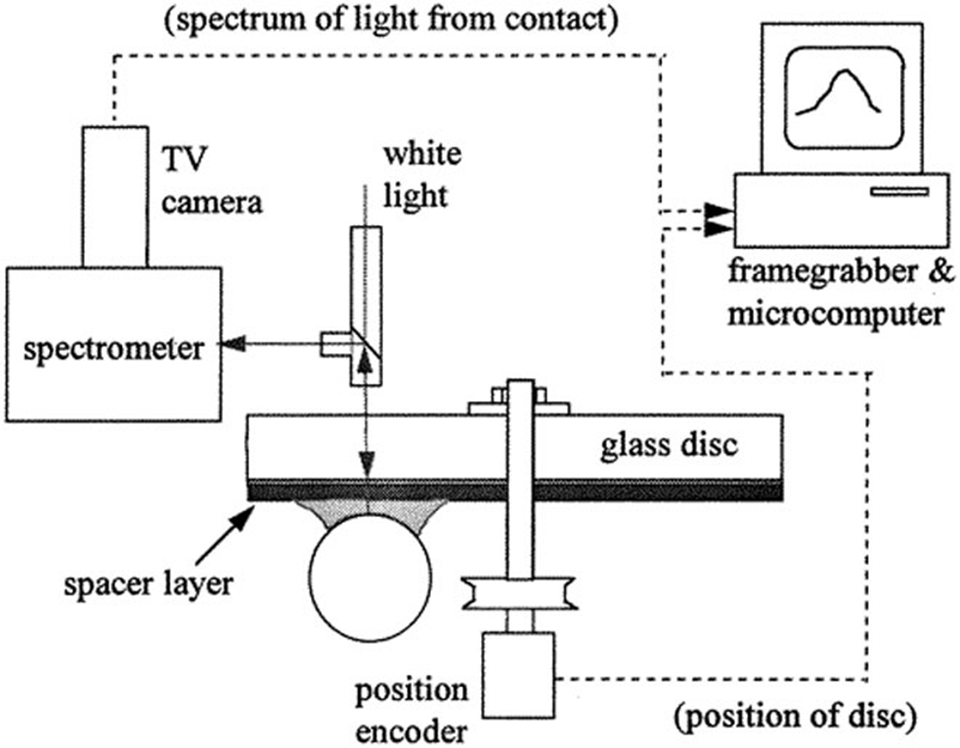
The variation of architectural parameters of PLL-g-PEG, including PEG chain length, grafting ratio [Lys/PEG-chains], and PLL backbone length, have been observed to be significantly influencing the lubrication properties of the selected tribosystem.
Influence of the PEG-chain length
![Enlarged view: Figure 5: The influence of the molecular weight of the PEG side chains on the coefficient of friction (squares; y-axis on the left hand side) and lubricant film thickness (circles; y-axis on the right hand side) was measured as a function of speed by means of MTM and ultra-thin-film interferometry. The test lubricants were aqueous buffer solution containing either PLL(20)-g[3.4]-PEG(2) (red symbols) or PLL(20)-g[3.4]-PEG(5) (purple symbols). The lines between data points serve as a guide for the eye. Ball = stainless steel (19 mm in diameter), substrate = silica, buffer solution = 10 mM HEPES (pH 7.4), polymer concentration = 0.25 mg/ml, load = 10 N, T = 25 °C.](/research/tribology/lubrication-with-polymer-brushes-and-gels/aqueous-lubrication/aqueous-lubrication-with-polymer-brushes/_jcr_content/par/fullwidthimage_1157192458/image.imageformat.1286.1354303792.jpg)
Influence of the grafting ratio [Lys/PEG-chains]
![Enlarged view: Figure 6: The influence of the Lys/PEG grafting ratio on the coefficient of friction (squares; y-axis on the left hand side) and lubricant film thickness (circles; y-axis on the right hand side) was measured as a function of speed by means of MTM and ultra-thin-film interferometry. The test lubricants were aqueous buffer solution containing PLL(20)-g[2.2]-PEG(2) (yellow symbols), PLL(20)-g[3.4]-PEG(2) (red symbols) or PLL(20)-g[5.7]-PEG(2) (grey symbols).](/research/tribology/lubrication-with-polymer-brushes-and-gels/aqueous-lubrication/aqueous-lubrication-with-polymer-brushes/_jcr_content/par/fullwidthimage_1027788804/image.imageformat.1286.618522713.jpg)
Influence of the PLL-backbone length
![Enlarged view: Figure 7: The influence of the molecular weight of the PLL backbone on the coefficient of friction (squares; y-axis on the left hand side) and lubricant film thickness (circles; y-axis on the right hand side) was measured as a function of speed by means of MTM and ultra-thin-film interferometry. The test lubricants were aqueous buffer solution containing either PLL(350)-g[3.4]-PEG(5) (black symbols) or PLL(20)-g[3.4]-PEG(5) (purple symbols).](/research/tribology/lubrication-with-polymer-brushes-and-gels/aqueous-lubrication/aqueous-lubrication-with-polymer-brushes/_jcr_content/par/fullwidthimage_666423446/image.imageformat.1286.117270909.jpg)
Other Work and Perspectives
The tribological properties of PLL-g-PEG coated oxide surfaces on the molecular scale are also under investigation with atomic force microscopy (AFM), in collaboration with external pageProf. Dr. Scott S. Perry’s group at the University of Houstoncall_made, with an aim to understand the lubrication behavior of this copolymer on the molecular scale.
References
- M. Müller, S. Lee, H.A. Spikes, and N.D. Spencer, “Boundary lubrication of oxide surfaces by poly(L-lysine)-g-poly(ethylene glycol) (PLL-g-PEG) in aqueous media: the influence of architectural variation of the polymers on the lubrication properties” accepted for publication Tribology Letters (Feb. 2003)
- S. Lee, M. Müller, J. Vörös, S. Pasche, S. de Paul, M. Textor, M.Ratoi, H.A. Spikes, and N.D. Spencer, “Boundary lubrication of oxide sufaces by poly(L-lysine)-g-poly(ethylene glycol) (PLL-g-PEG) in aqueous media” Tribology Letters in press.
- N.D. Spencer, S.S. Perry, S. Lee, M. Müller, S. Pasche, S. de Paul, M. Textor, X. Yan, and M. S. Lim, “Biotribological approaches to the lubrication of engineering systems “, 29th Leeds-Lyon Symposium on Tribology in press.
Collaboration
- external pageProf. Dr. Scott S. Perry, University of Florida, USAcall_made
- external pageProf. Dr. H.A. Spikes, Imperial College, University of London, Great Britaincall_made
Funding
- Council of the Swiss Federal Insitutes of Technology, TopNano21 Program
- US Air Force Office of Scientific Research under contract #F49620-02-1-0346