Nano-scale Morphological Gradients
In some applications, for example tribology, cell behavior, protein adsorption or the superhydrophobic effect, features in the nanometer range also play a crucial role. Morphological gradients with topographical features in the nanometer range can be generated via direct assembly of a nanoparticle density gradient by a simple dip-coating process.
Method
In this method, negatively charged silica particles are adsorbed onto a positively charged poly(ethylene imine) (PEI)-coated silicon wafer by means of electrostatic interactions. Since the adsorption is a kinetically controlled process, a particle gradient can be created by exposing different parts of the substrate for increasing times to a colloidal suspension. This is achieved by slowly immersing the substrate into a colloidal suspension of silica nanoparticles, leading to a gradual increase in particle density along the substrate surface (Fig. 1).
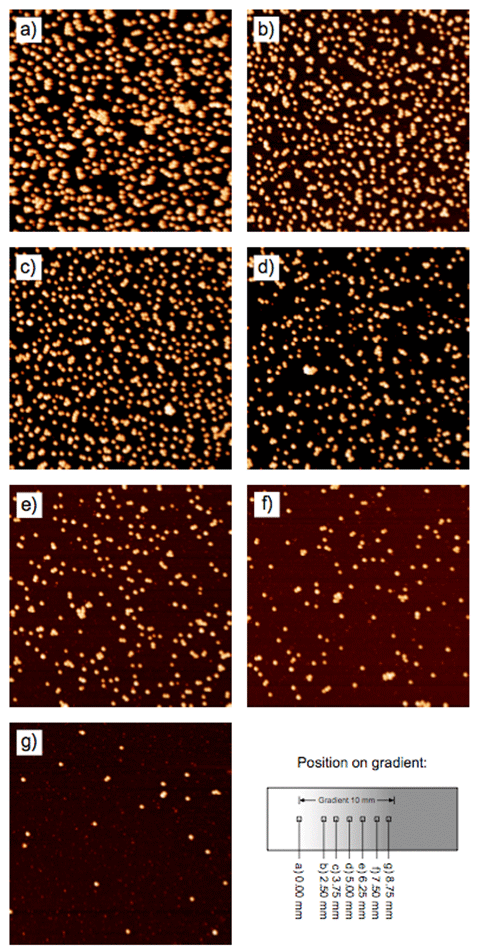
In order to increase the mechanical stability of the particle array and to adjust their shape the particle gradients can be partially sintered into the substrate at temperatures between 1075 and 1200°C. At such temperatures all organic compounds are burnt out, leaving nothing behind but a bare SiO2 nano-morphology gradient (Fig. 2).
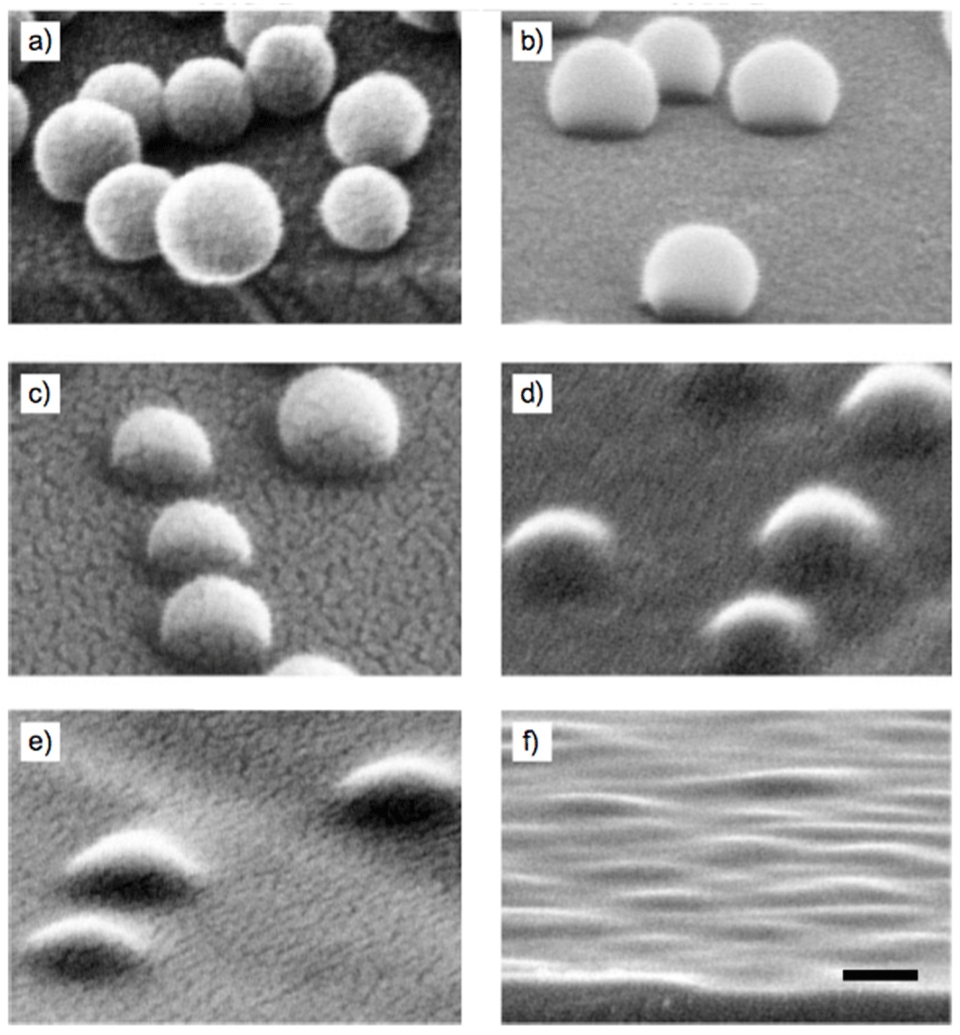
Cell Adhesion
Rat calvarial osteoblasts also adsorb gradually onto a nano-featured morphological gradient, as shown in Figure 3.
References
- Huwiler, C.; Kunzler, T. P.; Textor, M.; Vörös, J.; Spencer, N. D., external page Functionalizable Nano-Morphology Gradients via Colloidal Self-Assembly. Langmuir 2007, 23(11), 5929-593.
- Kunzler, T. P.; Huwiler, C.; Drobek, T.; Vörös, J.; Spencer, N. D., external page Systematic Study of Osteoblast Response to Nanotopography by means of Nanoparticle-Density Gradients. Biomaterials 2007, 28(33), 5000-5006.